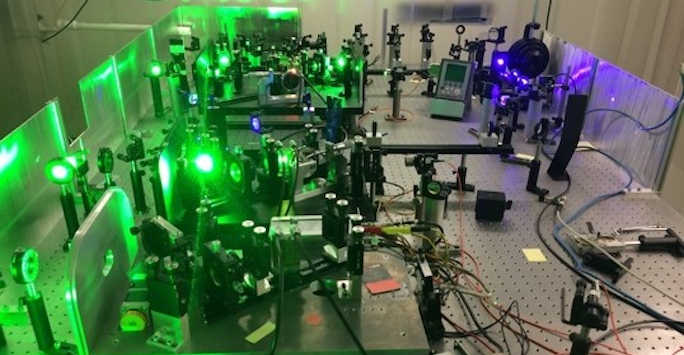
Laser spectroscopy of radioactive nuclei stands at the cornerstone of nuclear, atomic and laser physics. Many experimental techniques study the properties of nuclear decay, for example the energy of alpha or beta particles or gamma rays. In this way, nuclear structure is studied by looking at what changes in going from one nuclear state to another. Optical spectroscopy is an alternative approach, offering a probe of the nuclear states themselves.
To a first approximation, the electronic energy levels of the atom are arranged in a way that depends solely on the number of protons contributing to the nuclear charge - the element under study. Using lasers to excite the electrons between these levels tells us what the energy spacing is. High-resolution measurements are then able to reveal the hyperfine structure - the tiny shifts and splittings of these energy levels due to the properties of the nucleus. In this way, we are able to measure an array of fundamental properties, such as nuclear sizes and shapes, their magnetism and quantum spin numbers.
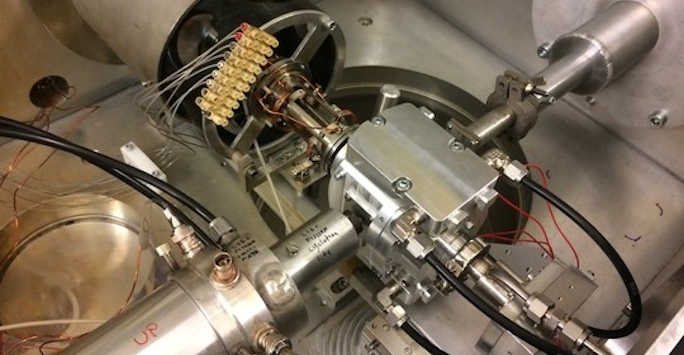
Our research is performed at international “isotope factories”: at the University of Jyväskylä Accelerator Laboratory, Finland; at ISOLDE, CERN; and at Canada’s national facility, TRIUMF, Vancouver; and at the GSI, Germany. Studying how nuclei change away from the valley of stability, and a stringent test of state-of-the-art models, requires measuring short-lived exotic nuclei - perhaps they only live for a millisecond. For this reason, nuclear reactions are induced using particle accelerators, and the products directly extracted into beams of radioactive nuclei. These travel at speed along extensive networks of evacuated “beam lines” where much manipulation takes place before spectroscopy is performed in-flight. In addition to spectroscopy, the unique “optical fingerprint” presented enables lasers to be used as a tool to purify such beams for single nuclear species.
Recent highlights include the first observation of an optical excitation in a trans-fermium element, nobelium (Z=102), published in Nature (Nature 538, 495 (2016)). This then enabled us to determine properties of both the nuclei, such as their size and shape (PRL 120, 232503 (2018)), but also their atomic (electronic) structure such as the first ionisation potential (PRL 120, 263003 (2018)). In ISOLDE, we have led studies of manganese (PLB 750, 176 (2015), PLB 760, 387 (2016)), zinc (PRL 116, 182502 (2016), PLB 771, 385 (2017), PLB 797, 134805 (2019)) and nickel (PRL 124, 132502), isotopes, mainly investigating how the proton and neutron energy levels change with neutron number, and in some cases the consequences for the “magic numbers”. In Jyväskylä (PRA 97, 042504 (2018)) where novel methods produce nuclei which are sometimes not available elsewhere, our group has recently upgraded the laser, optics and spectroscopy infrastructure, paving the way for the next generation of measurements.