QUASAR R&D at IPAC22 – Novel Accelerators
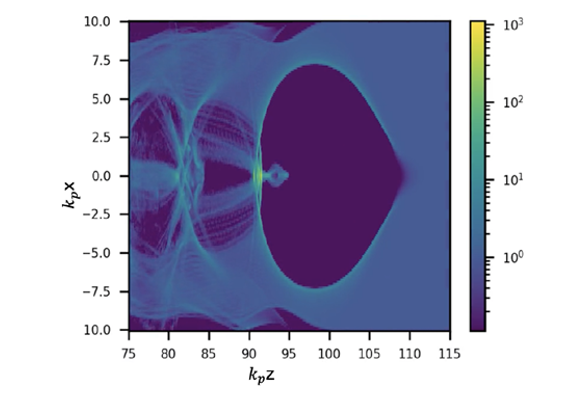
Within the novel accelerators section of IPAC 2022, the QUASAR Group contributed with a number of original research articles based on Particle-In-Cell (PIC) simulations. Below is a summary of the Group activities related to the design and development of novel accelerators.
The Facility for Advanced Experimental Tests II (FACET-II) at Stanford University will be a test bench for beam-plasma interactions. The QUASAR team simulated an experiment which is due to take place at FACET II, with the goal of producing electron bunches of ultra low transverse emittance. Tiny oscillations of such electron bunches lead to X-ray emission (betatron radiation) which can be used as a diagnostics tool. Using the OSIRIS code, an X-ray generation event was modelled.
The simulations showed that an electron bunch with a transverse emittance of 2 mm − rad and kinetic energy of 500 MeV, undergoes transverse oscillations of up to 1 micrometer elongation. [1]
The electron plasma density expressed as the ratio to the unperturbed gas density, shows a bubble of ions, surrounded by background electrons. Longitudinal electric field of 40 GV/m inside this bubble accelerates a bunch of electrons trapped inside. (Image credit: Monika Yadav)
When simulating beam-plasma interactions, ions are typically assumed to remain at rest. However, with light gases such as hydrogen, this assumption is wrong and may lead to inaccurate results for a long beam propagation range. In fact by stimulating ion motion the accelerated electron beam might be better focused. We have explored a focusing system made of quadrupoles and plasma lenses and. PIC simulations spanning a few centimetres have shown that in certain conditions ions collapse due to the strong electric fields driven by the accelerated electron bunch.
When this occurs, the electron bunch undergoes a radial compression. Our scheme proved a focusing effect 33% larger than that obtained with quasi-fixed ions. [2]
(Top) Electron density after the collapse of the ion column and (Bottom) The ion density. (Image credit: Monika Yadav)
As intense particle beams propagate in dense plasma or gas, femtosecond ionization effects play a fundamental role in the particle dynamics. This is usually ignored in comparison with the much stronger wakefield forces. The QUASAR Team carried out PIC simulations aimed to understand the ionization in a gas sheet and to prepare an experimental setup.
We modelled a 10 GeV electron beam with a charge of 0.5 nC and captured the ionization process. The results showed that ionized electrons may be directly trapped in the wakefield bubble along with the slow capture of background electrons. [3]
Gas sheet ionization monitor in development. Image credit: Nathan Cook (Radiasoft)
Plasma Wakefield Acceleration (PWFA) is a novel technique that is poised to make a step-change in particle accelerator technology. In the proof-of-principle AWAKE experiment at CERN, a 12 cm-long beam of protons is diverted into a narrow channel of plasma where it excites an electrostatic wave (a plasma wakefield) which can in turn be used to accelerate an externally injected electron beam to very high energy. Because of its length, the proton beam must first be broken up into shorter ‘micro-bunches’ by the wave in a process called seeded self-modulation (SSM), which in turn enhances the plasma wakefield. While most theoretical and computational works have considered SSM assuming a cylindrical symmetry, we have studied how this effect is influenced by the transverse asymmetry of the incoming proton bunch. Using 3D particle-in-cell simulations and an improved analytical model, we have investigated the behaviour of non-cylindrical beams undergoing SSM, and found a dependence of the growth rate (which varies with a ‘beam shape parameter’, ν) with the initial transverse aspect ratio of the beam, h. [4]
Variation of the beam shape parameter during the growth stage of the microbunching effect while the initial aspect ration is varied. (Image credit: Aravinda Perera)
Laser or beam driven plasma originating from nanomaterials may offer the possibility to accelerate electrons through extremely high gradients. This is due to the high plasma density which approaches that of metals. The QUASAR team has shown numerically, using the PIConGPU code, that UV laser pulses can indeed create longitudinal electric fields as high as 5 TV/m, just behind the pulse.
This may be used to accelerate externally injected electrons provided that injection occurs in the blue (negative) region of the longitudinal electric field Ey. The simulations showed that stable wakefield bubbles of 300 nm longitudinal size are formed through a dynamics of the background electrons, very similar to that found when IR lasers interact with gas-plasmas. This research supports alternative routes to design high-gradient compact accelerators. [5]
Longitudinal electric fields driven by a UV laser pulse in a carbon plasma whose limits are shown by the green rectangle. (Image credit: Cristian Bontoiu)
- Physical aspects of collinear laser injection at SLAC FACET-II “E-310: Trojan Horse experiment
Link: https://doi.org/10.18429/JACoW-IPAC2022-WEPOST041 - Simulating Enhanced Focusing Effects of Ion Motion in Adiabatic Plasmas
Link: https://doi.org/10.18429/JACoW-IPAC2022-WEPOST045 - Gas Sheet Diagnostics Using Particle in Cell Code
Link: https://doi.org/10.18429/JACoW-IPAC2022-MOPOPT066 - Modelling Growth and Asymmetry in Seeded Self-Modulation of Elliptical Beams in Plasma
Link: https://doi.org/10.18429/JACoW-IPAC2022-WEPOTK030 - An Effective-Density Model for Accelerating Fields in Laser-Graphene Interactions
Link: https://doi.org/10.18429/JACoW-IPAC2022-WEPOST043