Charge radii of exotic potassium isotopes challenge nuclear theory and the magic character of N = 32
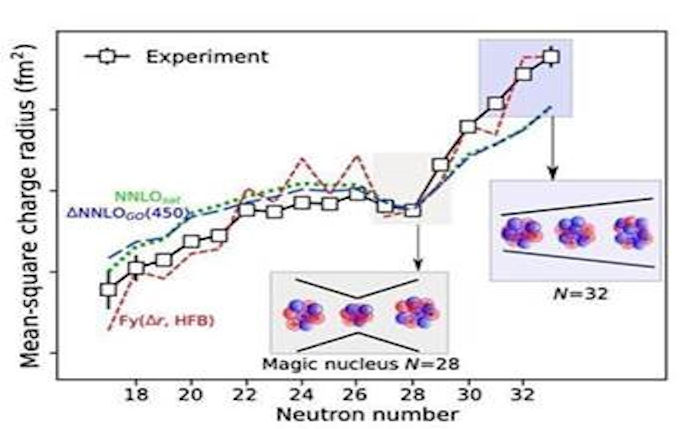
The size of atomic nuclei has proven to be a powerful probe for the nuclear force. The charge radius, which can be extracted from laser spectroscopy measurements, is sensitive to both the bulk properties of nuclear matter and also the subtle details of the interaction between protons and neutrons. Nuclei with unbalanced proton to neutron ratio (exotic nuclei) exhibit new phenomena and continuously test our understanding of the nuclear forces.
One example of these is the emergence of new magic numbers. The magic character of a proton or neutron number is reflected in a smaller size of the magic nucleus, compared to its neighbours (grey box). There are several well know magic numbers such as 2, 8 20 or 28. In the mass region of the potassium isotopes, 32 has been proposed as a new magic neutron number.
A measurement of the charge radius of 52K was achieved using the Collinear Resonance Ionization Spectroscopy experiment at ISOLDE, CERN. The results show that there is no sign of magicity in the evolution of the nuclear size across the N=32 neutron number (blue box). Furthermore, the experimental results were compared with state-of-the-art theoretical calculations. Two different theoretical models can be applied for the K isotopes. One is the coupled cluster method (CC), which is an ab initio method that can only be applied to light nuclei. The other is density functional (DFT) theory, which is highly successful in the heavier mass region. From the comparison between the measured and predicted changes in the mean-square charge radii it is clear that the calculations perform very well in predicting the general trend below the N=28 magic number, successfully taking on the challenge of modelling isotopes with unpaired protons and neutrons.
At a closer look however, it becomes apparent that the CC calculations fall short in predicting the steep increase in the charge radii of the neutron rich isotopes. The fact that two different potentials (blue and green lines in the figure) result in identical trends above N=28 highlights that issue may lie in the employed many-body methods. The Fayans DFT on the other hand, predicts the general trend very well, however, it overestimates the variation between the size of odd and even mass isotopes. These results therefore present a major challenge to theory.
This work has been published this month in Nature Physics by Agi Koszorús, who is a PDRA now working in our nuclear physics group. Agi is based at the JYFL Accelerator Laboratory, Finland, working with Bradley Cheal in leading our laser spectroscopy experiments at the IGISOL facility. These experiments give unique access to a comprehensive range of fundamental properties of a nucleus in its ground or isomeric state, such as the size, shape, magnetic moment and quantum spin. At the IGISOL, novel gas cell techniques provide beams of short-lived (perhaps sub-millisecond) isotopes for study, irrespective of their physical or chemical properties and therefore sometimes unavailable at larger facilities, like CERN. In recent years the Liverpool group have upgraded the laser system (largely thanks to a PPRP grant), all of the optics (from Consolidated Grant funding) and Agi is now helping us to upgrade the spectroscopy system. Meanwhile, being based locally, Agi has been leading our experimental efforts while international collaborators have been unable to travel due to COVID. Only this month, measurements of neutron-deficient chromium isotopes were performed, which will form the basis of the PhD thesis of one of our students. This is a region of the nuclear chart which is relatively unexplored, but that our experiments at the IGISOL can begin to address.
Experimentally measured changes in the mean-square charge radii of potassium isotopes (white squares) are compared to the predictions of state-of-the-art nuclear Coupled Cluster (green and blue) and Density Functional Theory (red) calculations. The grey box illustrates the trend of the charge radii across the neutron magic N=28, while the red box shows that the N=32 isotopes do not exhibit similar behaviour.